Leaded future
Lead was a crucial ingredient in our energy system for almost 100 years.
It was added to gasoline in the form of tetraethyl lead to improve the longevity, efficiency and performance of internal combustion engines. Unfortunately, it did not have the same effect on people inhaling the fumes.
Lead’s phase out began in the 1970s and is estimated to have resulted in $2.4 trillion in annual benefits, and 1.2 million fewer premature deaths, according to a 2011 UN study.
Now lead could make a comeback in our energy system, albeit in a more benign role.
Fission
Lead-cooled fast reactors use lead as a coolant for solid nuclear fuel rods.
Lead is abundant, relatively cheap and boils at over 1700°C, which enables low pressure systems and makes it possible to achive passive safety in compact forms. It does not react chemically with water, air or fuel, reducing the need for fire-supression systems, which are mandatory in the competing sodium-cooled designs.
The main problem with using lead is that it can corrode stainless steel. To solve this problem, either the exposed structures have to be replaced regularly, or they have to be protected against corrosion.
The Swedish company Blykalla considers their three alumina alloyed steels, which forms alumina oxides as a protective layer on the steel surfaces, as key innovation for making LFRs feasible. Their different steels are used for fuel tubes, the reactor vessels and pump components, respectively.
Lead’s neutronic properties ensure a very hard spectrum that makes this reactor type capable of breeding plutonium. It can also be run as burner for minor actinides, reducing the necessary time in geological storage.
We would need to reprocess the current generation’s spent nuclear fuel and dispose of the fission products, either using seabed disposal, deep boreholes or geological repositories, for example in salt domes, like at WIPP.
Lead-cooled reactors have a rather long history of research and usage, especially in the Russian military, which deployed 4 reactor cores (73MW) in prototype submarines and 7 in “Alpha Class” submarines (155 MWt). ~80 reactor-years experience could be gathered, with some painful lessons learned.
Today several companies in the West, like the Swedish Blykalla (formally known as LeadCold), Westinghouse or the relatively young, and well-funded newcleo, which acquired Hydromine’s IP in LFRs, are working on commercializing a design. Hydromine had developed some rather interesting solutions to make their design more robust, compact and affordable.
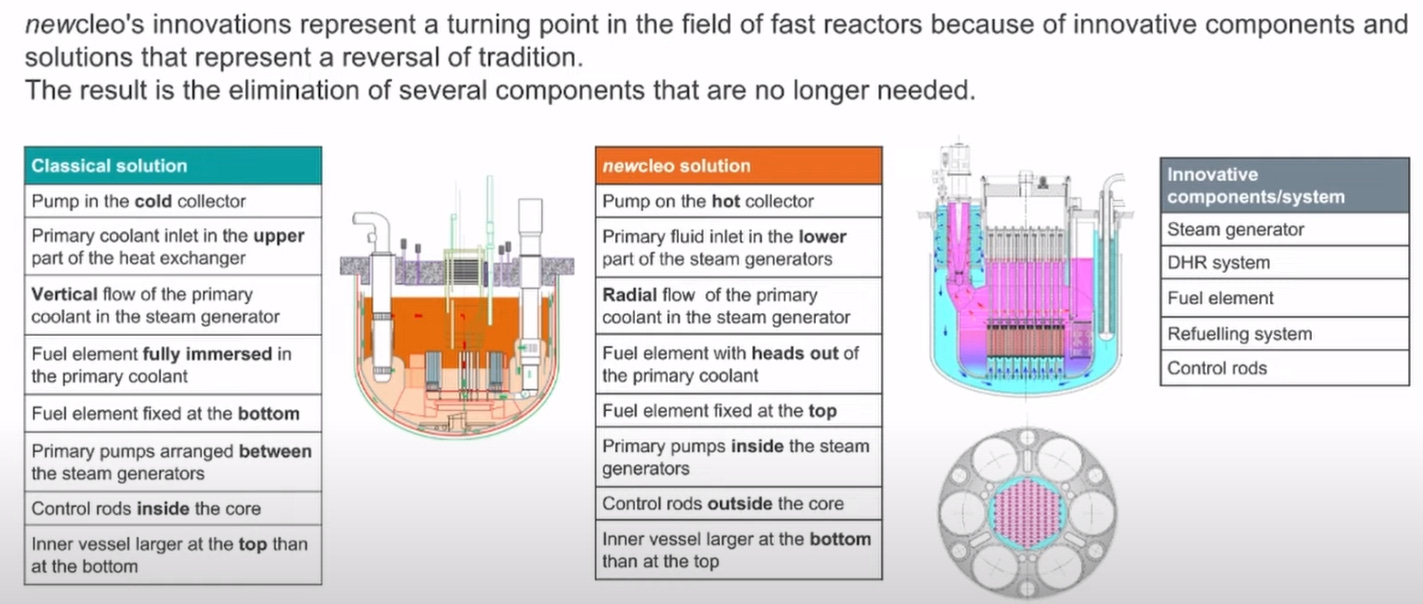
Especially in comparison to a large SFR, like the French Superphénix, the compactness of the LFR designs is apparent.
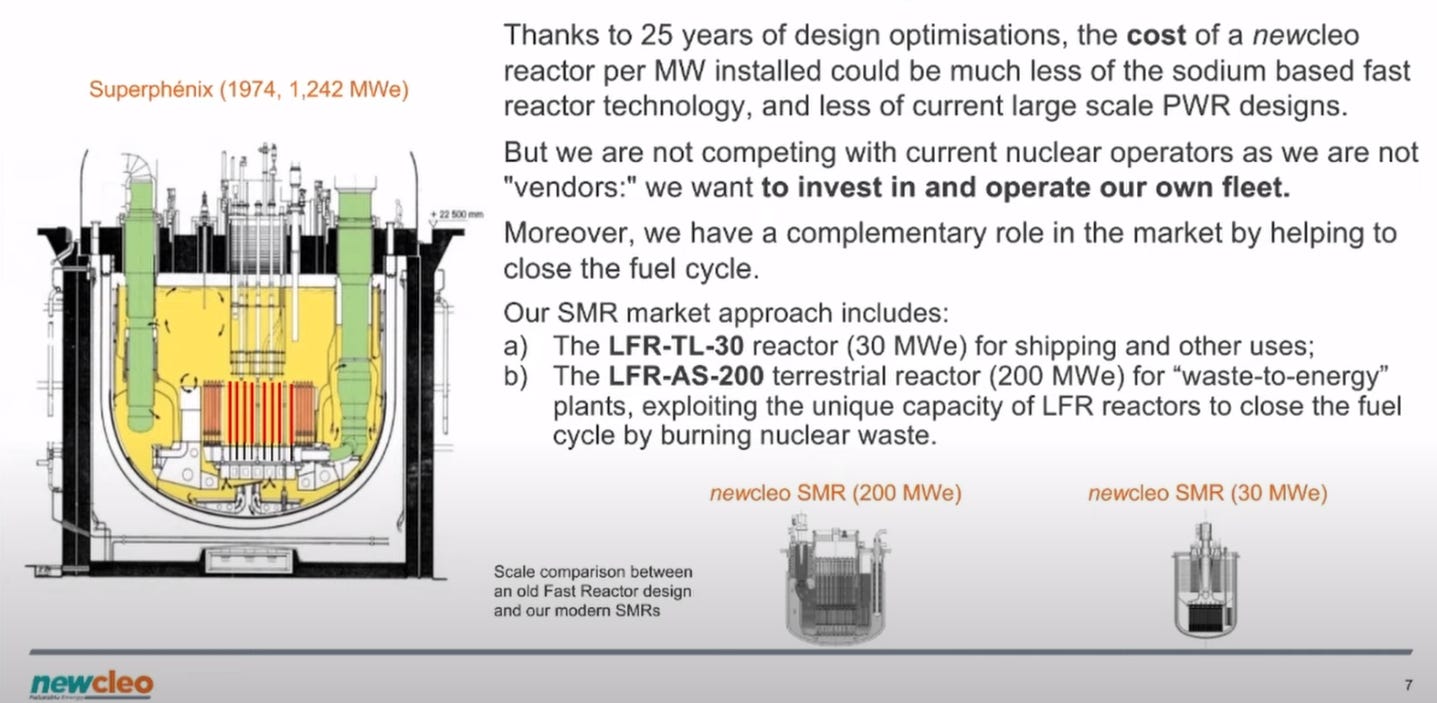
While newcleo is looking at “conventional” MOX fuel, Blykalla is working on nitride fuel, which features 40% more uranium per volume unit, which equals a 40% longer life for the fuel. Its melting point is > 1,500°C higher than the operating temperature, with seven times higher thermal conductivity in the fuel compared to oxide fuels. The hard spectrum of LFRs would also make them good candidates for using reprocessed fuel.
Curio is the only privat endeavor mainly focused on reprocessing fuel as their business model, that I am aware of. (Moltex or Exodys are working on fuel conversion processes for their chloride fuel salt reactors, but their main focus is the development of said reactors).
Curio is developing a reprocessing cycle called “NuCycle”, which recovers uranium for reenrichment, a mixture of transuranic elements from the spent-nuclear fuel of LWRs as fuel for advanced reactor types, and simulatneously isolates fission products for disposal.
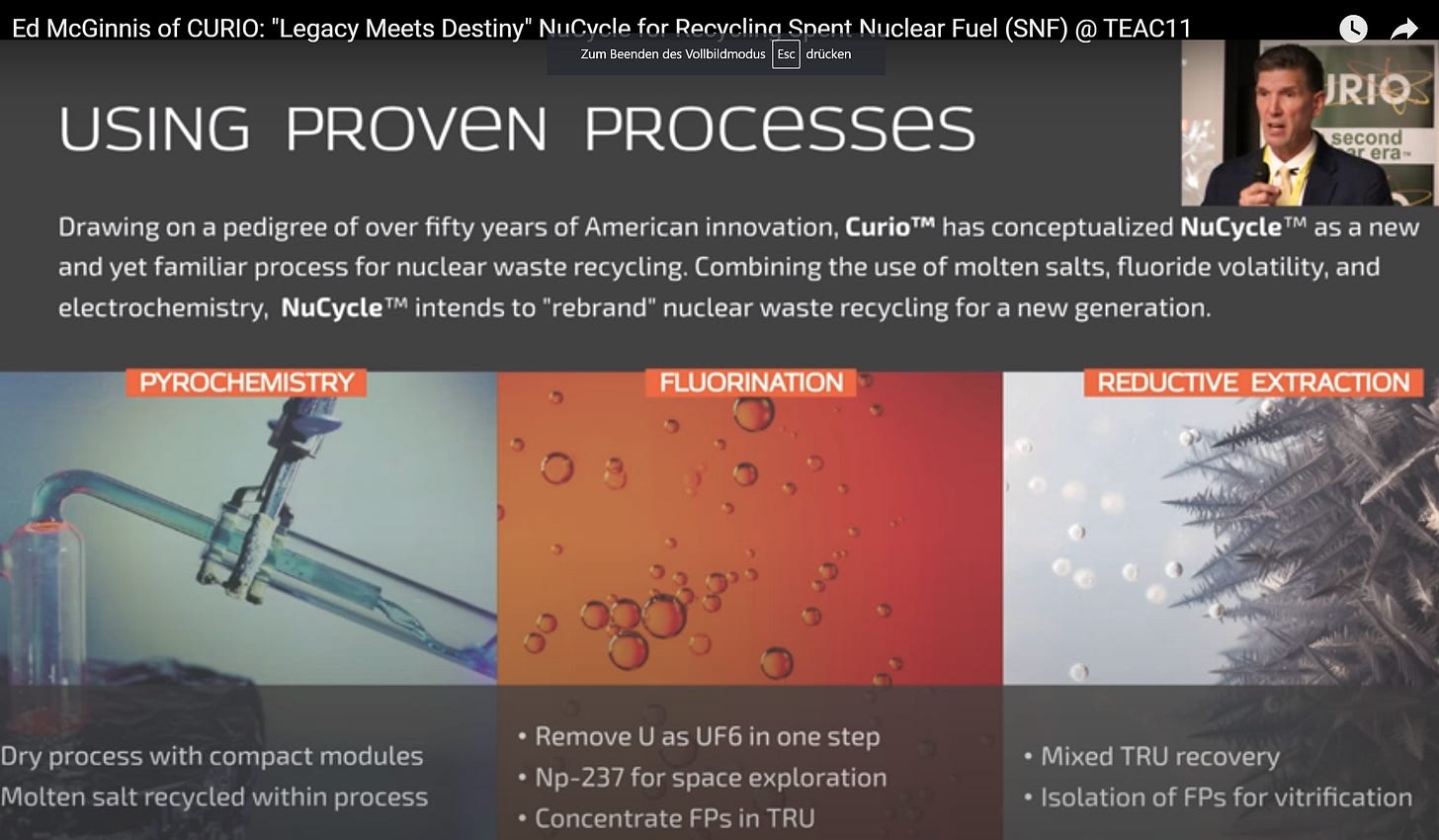
Depending on the mixture of the fuel, a LFR can act as a breeder or burner of plutonium and/or minor actinides. In equilibrium, a LFR would only consume natural or depleted uranium as make-up fuel and only discharge fission products. These need to be stored for something like ~300 years, before they are less radioactive than the uranium ore that was their “parent”.
Theoretically, there would be no need for enrichment facilitities.
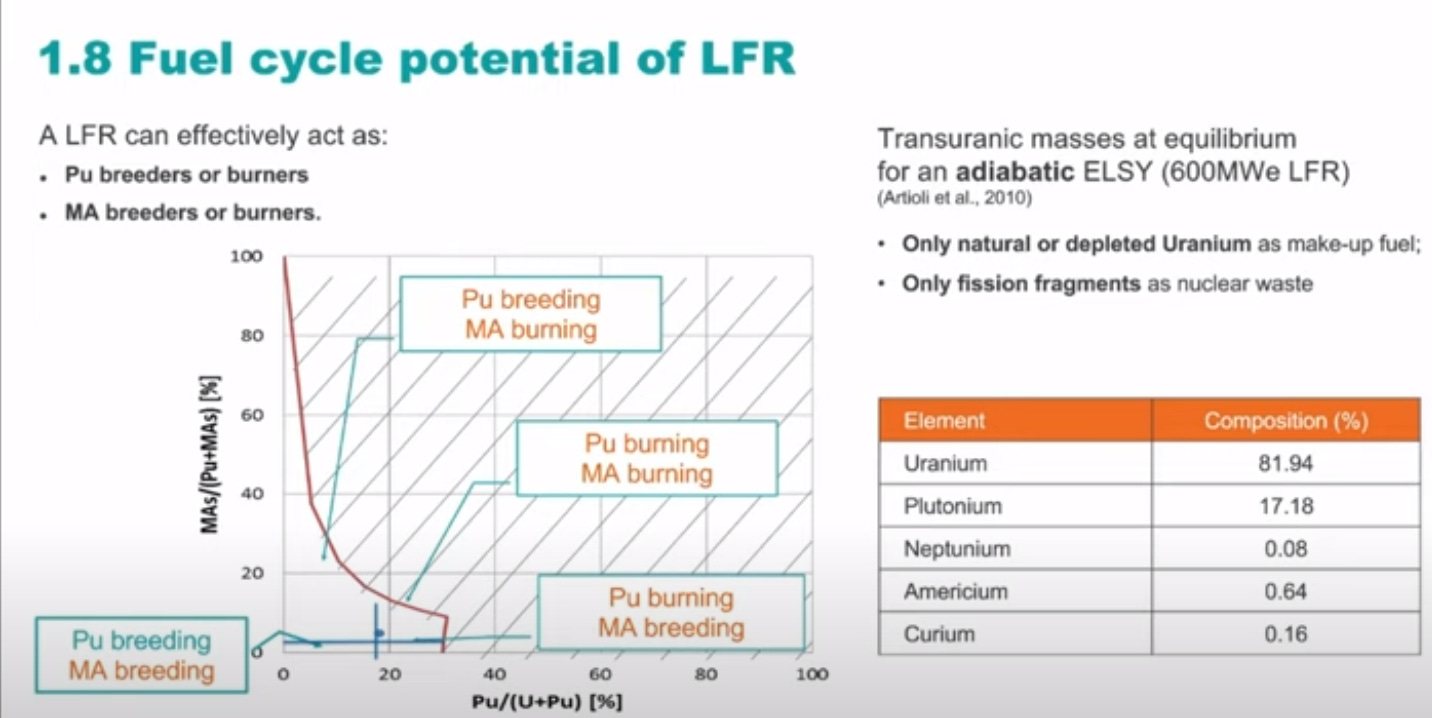
There are several known ways to store spent nuclear fuel or pure fission products. The only private company that I am aware of, which tries to work on fuel disposal is Deep Isolation.
They use standard drilling technologies for creating deep boreholes, into which the packaged material can be injected for safe and permanent disposal. This technique voids the need for a central repository. It might be often be possible to construct a geological “repository” under a power plant’s plot of land, which should reduce the need for transporting nuclear fuel considerably.
Russia is also working on a LFR design (BREST-300) to close their fuel cycle. Given that Russia is currently the only nation to have a robust fast power reactor sector with the sodium-cooled “BN-800”, their renewed interest in LFR technology gives proponents of this technology, as well as critics of the SFR technology, some validation for their positions.
A more radical and newer approach in LFR technology is pursued by Dual Fluid, a company started in Germany, but now relocated to Canada.
This company is looking at using molten, metallic uranium-plutonium fuel, which would get circulated through a core made of either refractory metals or siliconcarbide, and be cooled by molten lead.
Dual Fluid is stating a target operating temperature of approximately 1000°C, online reprocessing in larger units and a melt fuse to drain the core in overheating scenarios. Such a reactor would have extremely high breeding coefficients. The operating temperature is far above the level considered for other designs, which have to keep the temperature at ~500°C, to have enough safety margin in transient conditions, and to limit the corrosiveness of lead on the steel strucutres.
Rwanda has shown interest in this reactor.
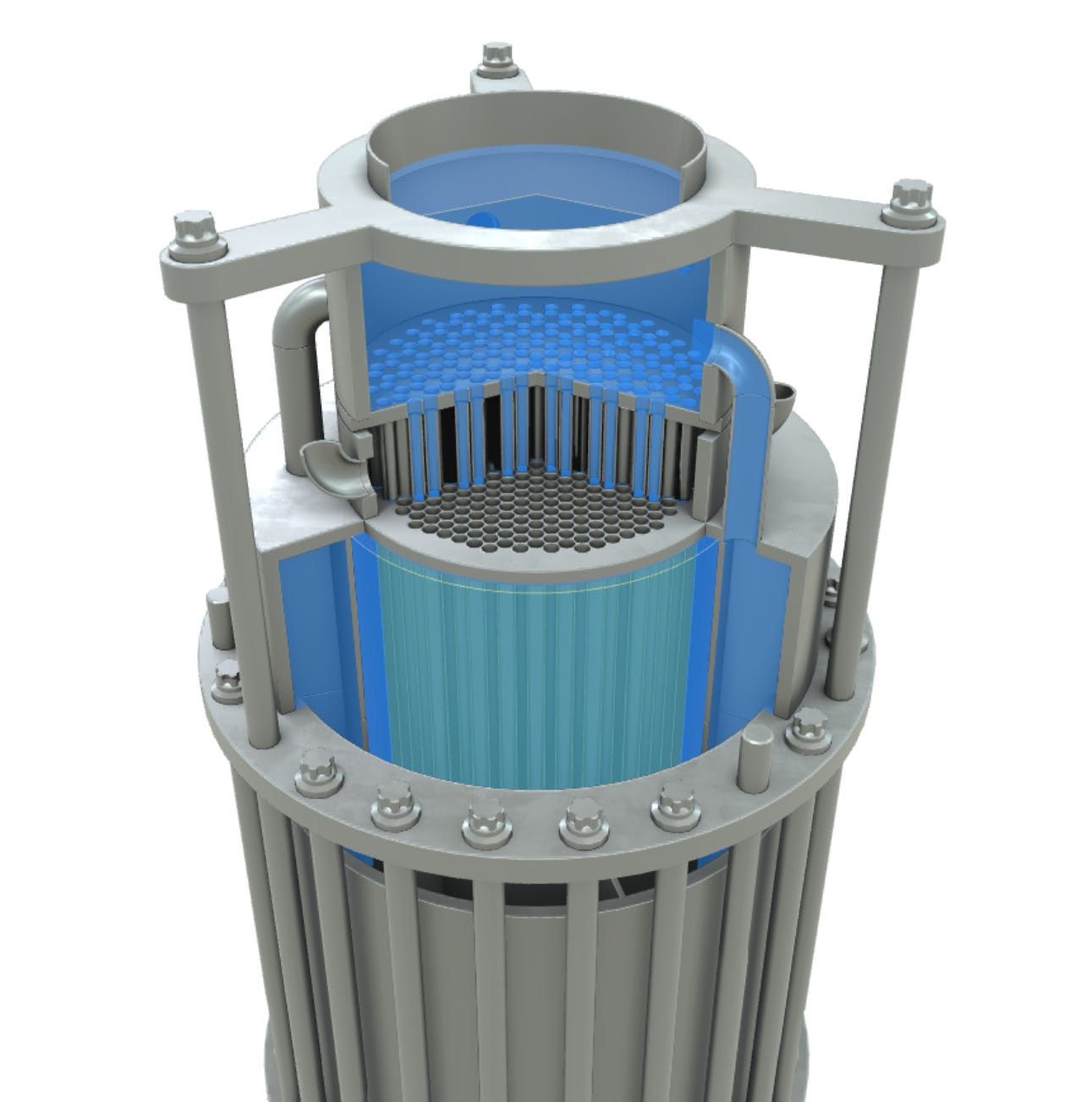
Fusion
Lead’s role in the future’s energy system is not limited to fission power.
Deuterium-tritium fusion offers the easiest path to fusion, with the most “benign” conditions for ignition. Unfortunately, tritium has a half life of only 12 years, so it is highly radioactive (really weak beta decay) and there is hardly any of it naturally. Fortunately, we know how to breed it from lithium-6, a stable isotope.
But just as with fission breeding reactors, the utmost care is necessary to ensure that enough neutrons are “reserved” for breeding and are reaching the fertile material.
The “first wall problem” has been a major reason for concern for the commercial viability of fusion devices, and thus a major topic for research. That’s because the first wall has to fullfil several critical functions simultaneously:
It has to absorb most neutrons to protect expensive components, like magnets from neutron damage and frequent replacement,
it has to be heat resistent, it is has to allow for a coolant to extract heat from the fusion process, and
it has to allow for enough of the neutrons to reach fertile material to replenish the tritium fuel.
There are several fusion reactor designs that rely on lead as part of a liquid first wall.
General Fusion is working on “magnetized target fusion”.
They want to create a vortex of molten lead by spinning it rapidly in a chamber. A plasma of deuterium-tritium is injected into the void. Using large pistons, the vortex is collapsed, compressing the plasma to fusion conditions. Lead absorbes the heat of the fusion process, protects the vessel from radiation damage and acts as coolant. The heated lead is used to produce steam in a heat exchanger and cycled back into the reaction chamber. Additionally, lithium is mixed into the lead an acts as breeding material for tritium.
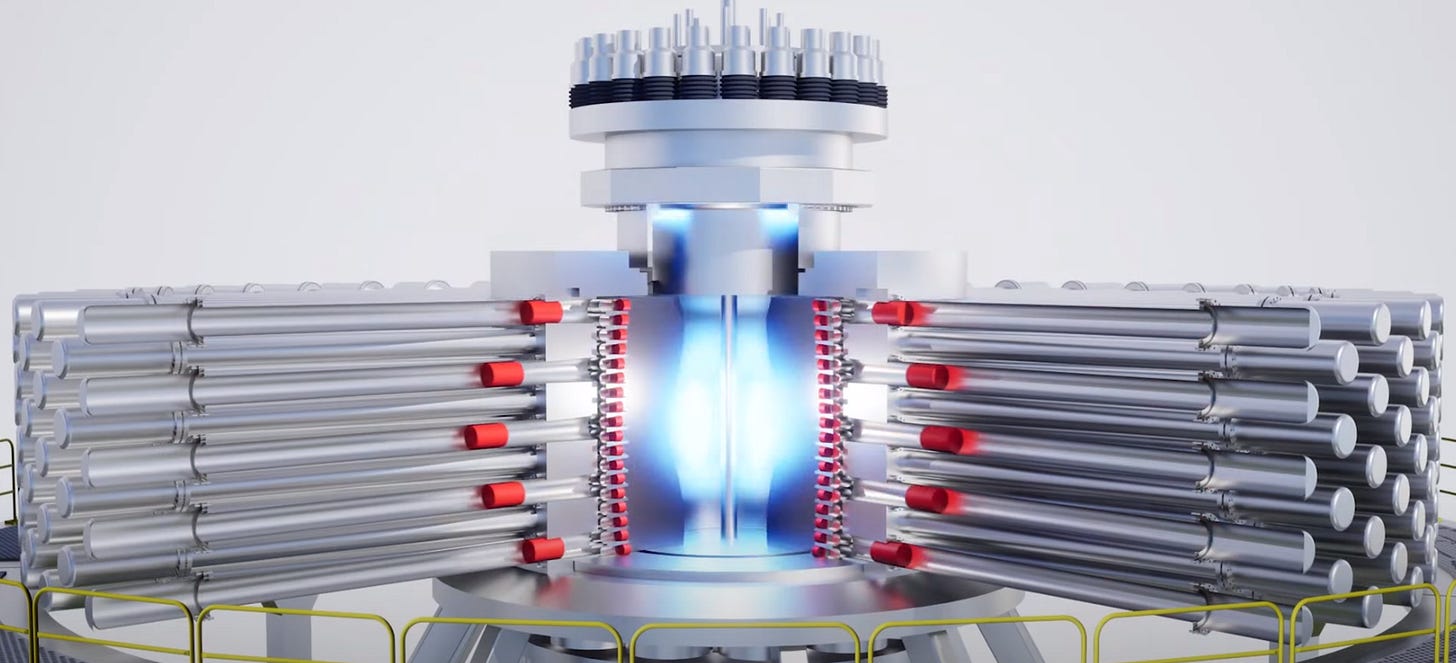
Zap Energy is working on the fusion device utilizing the z-pinch effect, where a current running through plasma creats a magnetic field that compresses the plasma. Hopefully up to commercially usable fusion conditions.
While this method does work in producing some fusion reactions, it is prone to plasma instabilities, which so far have prevented this approach from producing more energy by fusion than was needed to start the reaction. Zap’s approach is to induce different speeds in the plasma, which stabilizes it during the pinch.
They want to pump lead over a solid weir wall, to creat a well of lead. The lead at the bottom of the well is used as electrical contact to close the electric circuit of the z-pinch current. Lead absorbs most of the fusion neutrons, protecting the structural materials of the vessel. Just like with General Fusion’s approach, lead is also the primary coolant and mixed with lithium for breeding tritium.
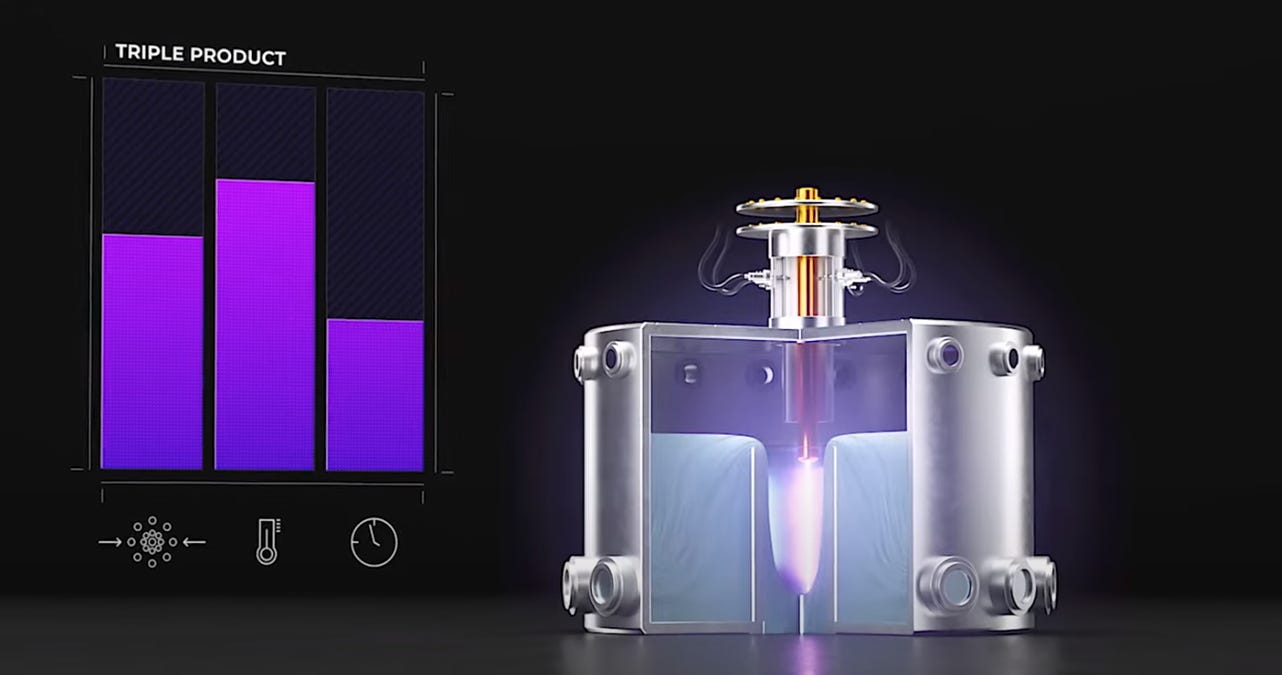
Lead has also a role to play in stellerators, fusion devices that confine plasma magnetically.
Renaissance Fusion, a European startup is working on revolutionizing this fusion device. Instead of relying on the geometrically highly complex 3D shapes of superconducting magnets, which have so far hampered the more wide-spread deployment of stellerators, Renaissance Fusion is changing the process from building the coils up by layering magnetic tape into complex geometric shapes to layering the super-conducting material on regular tubes.
After that, they use a laser to engrave the complex shapes of the magnet coils onto that tube, shifting the complexity from manufacturing to numerical optimization for finding the optimal pattern of the coils.
Thea Energy tries a somewhat similar route, where they use many, standardized planar coils to shift complexity from manucaturing and maintenance to finding optimal control algorithms for coils to shape the mangetic fields.
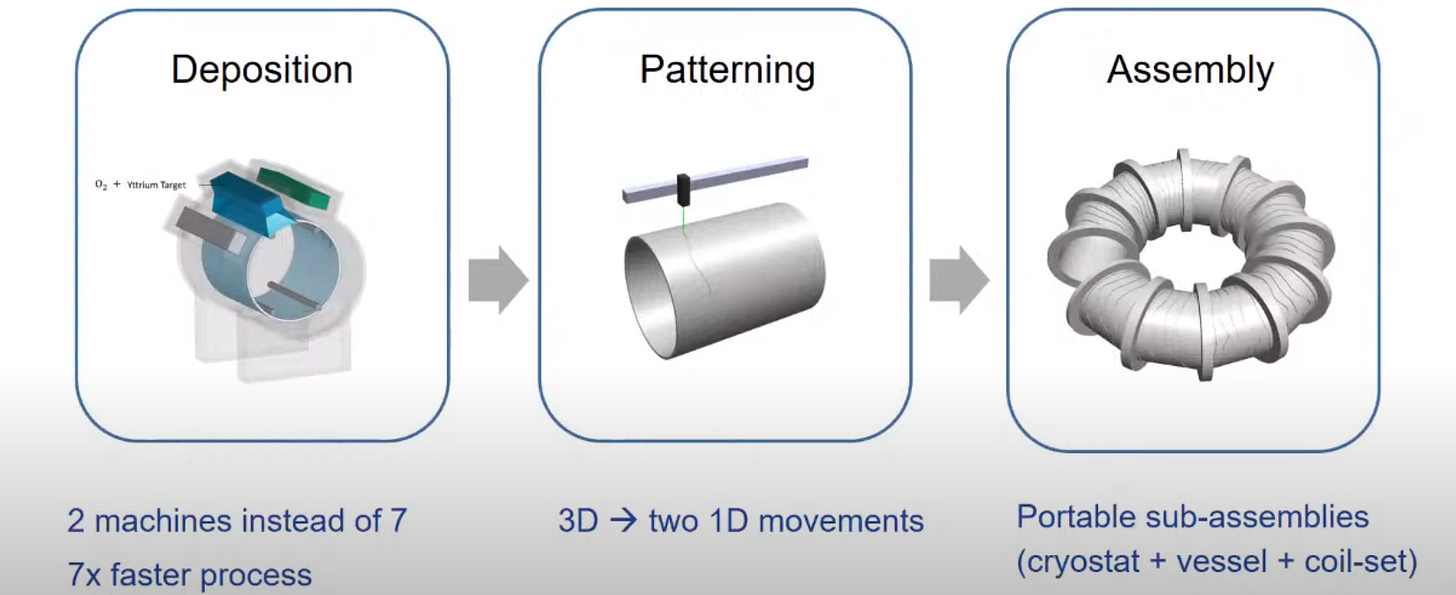
Inside Renaissance Fusion’s stellerator, they want use a thick layer of a mixture of liquid lithium and lithium-hydride. This acts as primary coolant and breeding material for tritium. A current running through the lithium and interacting with the magnetic fields, which confine the fusion plasma, forces the mixture onto the inner wall.
Renaissance fusion wants to embed lead in ceramic capsules, which would float on top of the lithium, facing the plasma. Lead acts as a multiplier for neutrons and moderator of the highest energy neutrons.
The combination of lithium-hydrides, which moderates the lower energy neutrons, and lead is supposed to decrease the necessary thickness of neutron shielding from 1.5m to 0.4m.
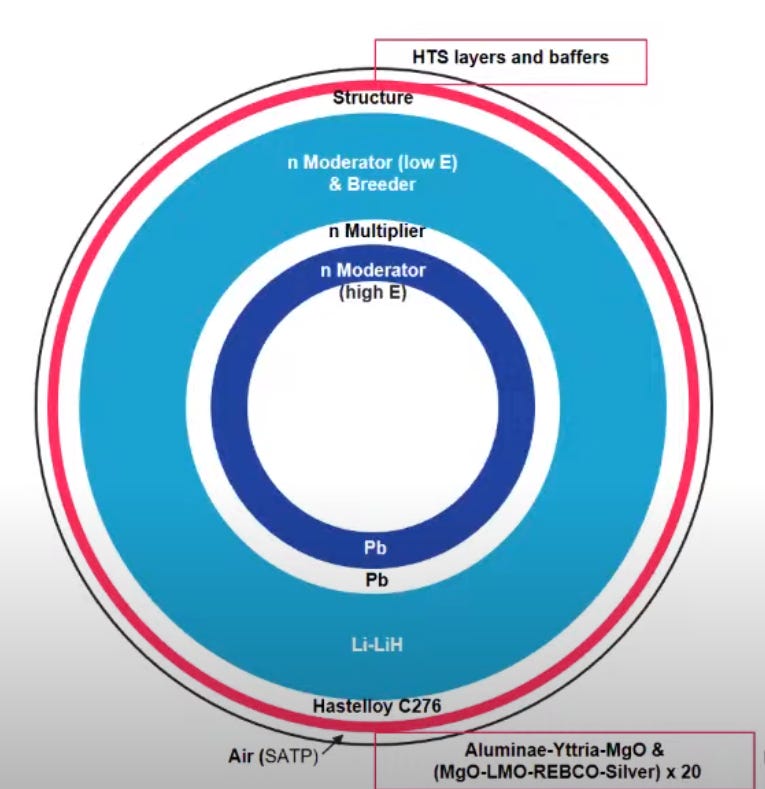
Summary
While we should cheer lead’s disappearance from fossil fuels and cars, it might be posed to contribute once again in our energy systems, though in a decidedly different role.