Inertial Confinement Fusion - Our best shot at climate change?
I brushed over the topic of Inertial Confinement Fusion, because I thought there were no companies developing it. Since then, I have learned that in fact several companies are pursuing this route to fusion.
So how promising is this route to clean energy on a global scale?
Why is there interest in Inertial Confinement Fusion?
The National Ignition Facility (NIF) at the Lawrence Livermore National Laboratory is the “flagship” of inertial fusion research. Its main purpose is research to make sure that US nuclear arms work as expected.
When I last wrote about it, it had reached about 1/3 of the energy necessary for ignition and for a time it did not look like they were making advances. But then, in August 2021, they announced a breakthrough, when it achieved ignition. A feat, which has since been confirmed by peer-review.
Scientists and engineers pursuing inertial confinement fusion have huge advantage compared to their colleagues working on other approaches towards fusion, like tokamaks or stellerators. They know that this approach does work and can produce net energy:
[T]here’s evidence that inertial-confinement fusion can produce net energy gain, not just in theory, but in practice. There’s just one problem: that evidence remains top secret. In the late 1970s and 1980s, a series of classified experiments, known as Halite and Centurion, that used nuclear explosives were conducted underground by the US national laboratories at Livermore and Los Alamos, respectively. We don’t know much about them because they’ve remained only partially declassified as they were conducted as part of nuclear weapons programmes. What can be guessed is that they involved indirectly driving an inertial-confinement implosion. The driver, in this case, was a massive dose of X-rays from a conventional nuclear weapon rather than X-rays indirectly generated by a laser. However, the X-rays took up the rest of the process as if it were laser fusion. […] What we do know about these secret inertial fusion tests is that they showed ‘… excellent performance, putting to rest fundamental questions about the basic feasibility of achieving high gain’ in inertial-confinement fusion. 1
The problem is: “What we don’t know is how close to the upper bound given by these experiments the laser energy needs to be before net energy gain can be achieved.” Today, there is a surprisingly large number of companies that are trying to answer this open question.
Update: 2022-12-30: Now we do know. Ignition and a Q>1 (thermal fusion power out / laser energy in) is possible. 3.15MJ for 2.05MJ laser energy.
Who is working on Inertial Confinement Fusion?
There are two general approaches:
Laser drivers
These companies try to use lasers to compress the fuel to start fusion:
Marvel Fusion: A German startup, focused on making the fusion of HB11 a reality. Its approach would not need a steam or gas turbine to covert fusion power to usable electricity, because most of the fusion energy is released as kinetic energy of charged particles. Marvel Fusion plans to deploy tailor-made nanostructured fuel pellets to take advantage of atomic-level effects that increase the rate of HB11 fusion reactions. It aims to build 500-2000MWe plants. They set up an industrial consortium in 2022 with Siemens Energy, Thales, TRUMPF & others for power plant development.
HB11: An Australian startup. The company is named after its intended fuel source, HB11. Their approach uses two lasers. The first one accelerates vanilla hydrogen to really high velocities, a second one is shot on a specifically designed coil. That laser ablates and ionizes surface metal, which induces a sudden voltage in the coil. This generates magnetic fields of kT strength. They hope to entrap the fusion fuel pellet with this magnetic field, after it has been hit by the block of accelerated hydrogen plasma. They are aiming for a first commercial plant in the 300-500 MWe range.
Focused Energy: An American-German startup that intends to use DT as fuel. They have a two-laser approach called “proton fast ignition”. The first laser fires a nanosecond–long pulse similar to NIF to compress the DT fusion fuel, then a picosecond beam from a second petawatt laser would strike a 1–µm–thick spherical foil around that fuel to generate very bright bursts of protons. This would “spark” the ignition of the compressed fuel. The two laser approach would - according to Focused Energy - allow resilience against capsule flaws, while NIF’s “indirect” approach is highly sensitive to target imperfections. The company aims for a 500-1500MWe plant design with a LCOE below $40MWh.
Innoven: A startup from the US. It is focused on developing KrF lasers and compressing tens of MJ laser pulses from microsecond-length pulses to the nanosecond scale with high optical quality, which is supposedly 150x less expensive than alternative approaches. Innoven intends to use a thick wall of molten FLiBe as moderator, neutron-shielding and breeding material. By going to high laser energy and large fusion yields, they estimate that a power plants needs only a firing frequency of 1 Hz.
Xcimer Energy: Has not released a lot of information. Their (rather minimal and slick looking) website states that they “will overcome long-standing obstacles to viable fusion power by integrating low-cost excimer [=excited dimer, my speculation is that they might be looking at KrF, like Innoven] laser technology with the HYLIFE reaction chamber concept[.] Xcimer’s laser architecture allows HYLIFE to be deployed in a simplified geometry and operated at lower repetition rate, significantly relaxing engineering requirements compared to conventional driver designs.” They aim at using DT fuel in the 1 GWe class.
Ex-Fusion: Japan’s first laser nuclear fusion company. From the Fusion Industry Association’s Report “The global fusion industry in 2022” it can be gleamed that this company intends to use DT fuel, but not much more. The website is also not really informative.
Kinetic drivers
These companies try to use kinetic energy to start the fusion process:
First Light Fusion: A UK startup. It uses an electro-mechanical driver, also known as railgun, to accelerate a projectile into a target in free fall. The target is designed to amplify the pressure generated by the one-sided driver in the DT fuel. The fusion energy is emitted mainly in the form of neutrons, which are captured in a rain of liquid lithium with natural isotope balance. The liquid lithium is supposed to function as neutron shield for the structural materials, as absorber for the huge heat loads and as breeding material for tritium. First-light fusion states: “Whilst we believe that a projectile system offers the best path to cost-competitive power production, this is not the only path available to us. Any system able to produce the required pressure on one side of the target could be used as an alternative. A high-energy laser, for example, would be able to do this. If laser costs were to fall substantially, First Light could benefit by developing an additional driver option using such a laser with our advanced target designs. The benefits of our simpler plant concept would remain the same, and the target design would still be the key enabling technology to make one-sided drive work.” They are aiming for ~150MWe plants firing once every 30s and costing less than $1G per plant. Despite that capital cost, CEO Hawker estimates that ultimately a LCOE of $25/MWh is possible. The approach has produced its first neutrons.
NearStar Fusion: is a rather recently funded company, which is developing a new pulsed approach to fusion called Hypervelocity Gradient Field Fusion (HGFF). Fusion fuel inside a metallic liner is accelerated with a rail-gun and fired through a magnatic coil, where it is raidly crushed by the magnetic field to compress and heat the fuel enough to spark fusion. The released energy is absorbed by molten FLiBe covering the walls of the reaction chamber. The company aims for approximately 50 MWe plants with the goal of modular scaling up to gigawatt class production. The approach itself could be iterated on to get to plasma conditions suitable for different fusion fuels. Their approach could also be used as engine for space travel.
HyperV: HyperV is pursuing the Plasma Jet Driven Magneto-Inertial Fusion (PJMIF) approach. Plasma guns are located around a spherical reaction chamber. The plasma guns fire deuterium-tritium plasma into the center of the reaction chamber. After a small delay, a plasma of heavy ions is also shot towards the center. The shots are timed so that two collapsing plasma shells are formed. The fusion fuel shell collapses to form a sphere in the center. The heavy ions arrive delayed. They are supposed to symmetrically compress and heat the fuel enough to start fusion. The reaction chamber is cooled by a liquid first wall containing lithium, which protects the solid sphere and the plasma guns from irradiation damage. Tritium is bred from the lithium in the liquid wall. This approach seems to be unique among the ICF approaches in that it does not use solid targets.
Challenges
Despite an “existence” proof and much enthusiasm, there are still major technological challenges. Let us compare the needs of one of the companies, Focused Fusion, as publicized in an interview, with some information about NIF from a rather recent book and get through the obvious dimensions of what is needed for ICF to work.
This is not to pick on a particular company, on the contrary, I believe they have an excellent staff (Markus Roth, Todd Ditmire, Pravel Patel), a lot of IP (for example all of National Energetics’, which built a 10TW laser for the Czech republic), good public communication and I believe they have one of the more promising approaches. I want to take them as example, because they have been transparent about their needs. That makes at least some ballpark comparisons possible.
Fusion Gain: What would a plant need in terms of gain, the ratio of power output to power input? Consider that gain is measured in terms of fusion energy output divided by laser energy input. There are additional conversion efficiencies, from fusion energy to electricity and from electricity to laser. Because a fusion power plant makes money by selling electricity (or heat), the complete conversion chain has to make economic sense. For economical fusion power, “[w]e need a fusion gain of 100, which is 100× the fusion energy of the laser[.]"2 The breakthrough of August 2022 at NIF achieved a gain of 0.7. That leaves us with a gap of roughly 100.
Lasers Efficiency: The heat flux inside the reaction chamber ultimately limits how much energy each fusion target is allowed to emit.(A good analogy might be a combustion engine, which also works with repetitive “explosions”. You cannot just make the explosions more violent without damaging the engine). Given that there is a need for 100x gain, an upper limit on the maximum power output3, a lower limit on how much laser energy is needed to spark the fusion process (again, in a conventional engine, you need a specific mixture of fuel and air to run it) and how much you can cool the lasers, you have a bound on the laser conversion efficiency that you need to meet. To put it mildly, NIF is not terribly efficient: “[O]f the 400 megajoules drawn from the grid, just half a per cent (1.8 megajoules) currently make it into the laser beam.” 1
Firing Frequency: How often would that laser have to fire? “Ultimately, you need a laser that is not only very efficient, but that fires at a high repetition rate, at least 10 shots a second.”2 How often does NIF fire? “NIF’s laser currently manages 400 shots per year. […] With the current technology on NIF, [firing at 10Hz] is impossible to do without breaking the laser. The optics need hours to cool down after each shot.” 1 That is a firing frequency of 0.00001Hz, leaving quite a large gap of roughly a factor of 1,000,000.
Laser Power: How much energy do you have to “squeeze” into each shot? While the “compression” laser in Focused Energy’s approach will be similar to NIF and use “long” laser pulses (~10-9 s) with “low” peak power (~1015 W/cm²) but a lot of energy per pulse (~106 J), albeit at a much higher repetition rate than NIF is capable of, the “ignition” laser will need far higher peak power and far shorter laser pulses. Based on results from Livermore, it is believed that 15 kJ worth of highly accelerated protons are required as “fusion spark plug”. “[W]e have demonstrated in trials that we can accelerate protons with an efficiency of roughly 10%, plus or minus, in the energy range we require. As a result, we require a 150–kJ short–pulse laser".2 The HAPLS (High Repetition-Rate Advanced Petawatt Laser System) is a LLNL designed laser that was commissioned in 2017 in the Czech Republic as part of a pan-European Research initiative called ELI (Extreme Light Infrastructure). This laser is designed for short pulses (~10-15 s) and high peak power (~ 1021 W/cm²). But it is currently “only” capable of 30 J per pulse. Which leaves a gap of a factor of ~5000.
Target Production: Given that commercial fusion will need a high firing frequency, a plant will need a lot of targets. “So if a fusion power plant runs at 10 Hz, 10× a second, you need 900,000 targets a day”2 But today: “It takes Livermore an order of months almost to build a perfectly shaped target”2 This leaves us with a gap of a factor of 10,000,000.
Target Costs: ICF needs highly sophisticated targets. “For NIF, the cost of these [are] hundreds of thousands of dollars[.]”1 These costs will have to come down to “just a couple of cents to make commercialisation viable.”1 That leaves us with another gap of a factor of roughly 1,000,000.
Tritium Production: All fusion plants that work with DT fuel, which is by far the easiest fusion fuel to ignite, require tritium. There is virtually none in nature. This means, that the power plants will need to breed tritium. Therefore, they will require enriched Li (except for First Light Fusion, which claims to get around the enrichment by using pure Li as coolant) and shower it with fusion neutrons. Some serious engineering will have to go into the enrichment plants and the breeding part of the fusion plants. The liquid first wall approaches seem to have a clear advantage in that regard. Companies trying to “skip” DT as fuel to go directly to HB11, will need to achieve approximately a 10x higher plasma temperature.
Conclusion
The comparisons above have to be taken with more than a grain of salt, though. NIF was commissioned in 2009 and laser technology has come a long way since then. “[T]he flashlamps that energise NIF’s 192 laser beams have been surpassed by diode-energised lasers. The newer diode lasers are around twenty to forty times more efficient and produce less waste heat.”1 They are also able to fire more rapidly and have fallen astonishingly in cost.
But while they can fire at a sufficient rate of 10Hz, they’re a long way from delivering enough energy with each shot. Of course, there are “bleeding edge” technologies, like LLNL’s plasma optics which could make lasers with 1000x peak power possible, and I am sure, a lot of people are working on making the necessary lasers possible (for example by improving the cooling).
There are also examples of precision-engineered products that are produced in the millions and cost only cents - like rifle ammunition. Additionally, Focused Energy’s approach is thought to be highly resilient to imperfections in targets, which should make the target production less demanding than it is for NIF.
But I think it is still fair to say, that the challenges that developers of inertial confinement fusion are facing are gigantic. Scaling the laser drivers and target production from the lab-scale to grid-scale, commercial fusion power will take a lot of innovation. And probably a lot of time. As always, I applaud the enthusiasm of the researchers and founders in this field, and I think it is exactly the right thing to do to fund research at the frontier of the possible via public-private partnerships.
But pondering on the scale of the technological challenges and on what is needed to make a dent in climate change, it seems obvious to me, that regulatory reform for fission plants in terms of licensing their designs and siting, is the less risky path to providing the tens of TW of clean, firm power that a prosperous world will need by mid-century.
I would like to remind you, that in the 1960s the world was able to build 1GW-class fission plants in about 4 years for far less than a $US1G in today’s money. And that is without the optimization that factory-production of for example floating nuclear plants could bring, without robotics and mostly without anything you would recognize as computer.
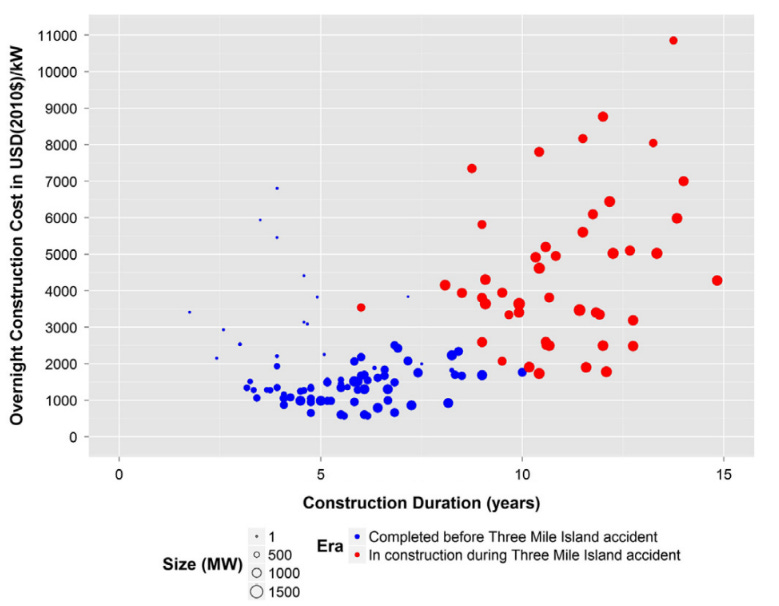
Lasers in the form of Uranium enrichment facilities, might play a pivotal role on that energy path as well. They might also be applicable for other isotope separation processes, like for Li-7 (for advanced fission reactor coolants), Cl-37 (helpful for fast molten-cholride salt reactors) or N-15 (used in some nuclear fuels, envisioned as coolant) enrichment.
A nice synergy is that the “tail” of Li-7 enrichment for fission plant coolants is exactly Li-6, which is needed for the DT fusion approaches. And obviously, the cooling system itself, material issues, radiation control, instrumentation, etc. are quite similar.
I hope fission power plants engineers can aid their scientist colleagues working on fusion, while the world moves towards a low-carbon, low-impact, high-energy, high-prosperity world. It’s the goal, we all should be working towards.
Turrell, Arthur. The Star Builders: Nuclear Fusion and the Race to Power the Planet. Orion. Kindle-Version.
Interview with Todd Ditmire, one of the founders of Focused Energy.
That is only true under the assumption, that the laser fusion approach needs a specific geometry and there are no breakthroughs in guiding “free” lasers. Project PACER, having a different driver, namely a thermo-nuclear bomb, and reaction chamber geometry, has quite different limits on the maximum yield per explosion! For more information, see: Long, F. (October 1976). “Peaceful Nuclear Explosions”. Bulletin of the Atomic Scientists. 32 (8), Section “Generation of Electric Power”. Not wanting to use nuclear fission weapons to drive fusion for power production purposes, might have been what sparked John Nuckolls’ interest and led to the concept of ICF in the first place. ↩︎